 |
|
 |
|
Work Programm
1. Excellence
1.1 Long term vision and targeted breakthrough
There is a real societal need for robust fast nanoscale information processing that will operate at room temperature. Based on our preliminary work, COPAC long-term vision is to firmly establish a new paradigm, and hardware and software technologies for the implementation at room temperature of ultrafast and complex parallel logic operations on arrays of quantum engineered nanomaterials that are addressed and read macroscopically.
COPAC will combine multidisciplinary experimental and theoretical breakthroughs to establish and validate novel hardware technologies for parallel and non-Boolean non-linear information processing that are based on pioneering multidirectional optical detection in arrays of purpose designed/tailored nanomaterials. We propose innovative high-risk nonlinear coherent multidimensional spectroscopy schemes that will combine the use of optical addressing by short laser pulse sequences with spatially resolved optical readout capabilities in order to demonstrate an industry standard certified prototype geared for unprecedented levels of speed, density and complexity in information processing.
COPAC builds on the new capabilities provided by the hardware that it will develop, to initiate new algorithms and new kinds of mathematical and computational approaches that are essential operations in large volume data analysis, data compaction and logic synthesis. These are enabled by the parallel processing of logic functions of multivalued variables with mission dedicated compilers and algorithms.
COPAC is based on the novel multidimensional ultrafast spectroscopy that has not yet had industrial applications. Preliminary experimental results demonstrating the application of the foundational principles to experimental systems including solid state arrays, are available. COPAC will made concrete steps to bring its results to a technology.
COPAC aims to realize parallel logic at room temperature at petahertz rates on ensembles of tailored nanosystems (supramolecular and quantum dots) in solution and in condensed phase arrays of increasing complexity.
The COPAC partners represent a broad range of disciplines in physics, chemistry and optics and in computer sciences as well as in nano-products manufacturing, that are needed for the success of this synergetic project (see table 3.3). We are effectively complementary and have collaborated before in various combinations in projects on different aspects of molecular and supramolecular logic. The partners have been recognized for their respective scientific and technical capabilities. An ERC award to EC(P3) and a Rita Levi Montalcini award to BF (also at P3) are recent examples of such recognition. The ability to empower new and high potential actors is also demonstrated by the fact that of the four early stage researchers, MaS, DHM, EC, BF, now being brought together in this project, two were trained and have developed their careers initially within our work on logic.
There is a significant difference between what we are proposing and quantum computing. COPAC operates with observables that are orthogonal to each other as further described in the section 4.1-P2-RL. A wave function is characterized by both amplitude and phase and both can vary continuously. The loss of phase due to the environment is often the faster relaxation. There is also an essential difference with classical stochastic molecular computing, related to a probabilistic finite state machine, where logic variables are encoded in the populations (= probabilities) of the molecular states, meaning the amplitude squared.
In COPAC we use a significantly larger set of observables: It takes N2 independent Hermitian matrices, each one of which is an observable that can be measured, to fully characterize a discrete N state quantal system [5,6]. COPAC will perform classical logic on a quantum engineered array that operates in parallel on N2 real valued variables instead of on N variables if only probabilities are followed. Our preliminary results are an explicit experimental demonstration for N =4 [2] and N=5 [4]. In COPAC we aim to realize significantly larger values of N. We will proceed in stages. In a given space direction we aim to increase to about N =12 states. Reading in many space directions (figure 1) we aim to reach N values of at least several dozens, see the theory developed in [2]. In an array of coherently coupled units we expect to address several hundred states. Beyond that point the ability to read reliably is limited by experimental resolution.
COPAC will develop a physical implementation of parallel multi-valued classical logic on its quantum engineered hardware, i.e. computing in parallel the outputs of a multivariate logic function, f(Xl), for all possible values of its inputs, Xl, (Fig.1a). The function to be evaluated is determined by an ensemble of M optically addressed, photoactive nanostructures (= nodes). At any point in time the function f(X) is measured as the full polarization, P(t,r), of the ensemble at position r of the detection screen (Fig.1b). The signal exists only at such directions κl that fulfil phase matching (PM) conditions, (Fig. 1b). The signal is the sum over the contributions of individual nodes located at position rm. By measuring emission in different PM spatial directions, Kl, we resolve the function into its components, Pl (Fig.1b), , an expression very much analogous to a discrete Fourier transform. l is a triplet of multivalued integers. Selection of lasers intensity controls the range of values for each integer. Using weak lasers and so operating to lowest order in perturbation theory limits the range to -2 to 2, leading to five-valued logic variables. (Compare to Boolean variables that are two valued).
The experimental outcomes are spots located at
different spatial positions on the detector (Fig.1b).
Each PM direction corresponds to a grating produced
by the wave vectors of the laser pulses. A particular
direction is that of the echo as used in 2-dimensional
photon echo spectroscopy (2DPE). [1,4,5] We will
use three pulse sequences (Fig. 1b). For a given
system, the function P(t,r) can be tuned by varying
the amplitude and polarization of the pulses and the
delay between them, (Fig.2a). Outputs mapping
options are: a. integrate the emitted signal over
time, b. measure frequency resolved maps in each
emission direction or c. Do a and b for increased
parallelism, see section 4.1 P3-EC, figures 3.3 and 3.4. When frequency resolved maps are measured, the spectral
decomposition of the logic function is equivalent to a molecular decision tree [1], as is shown in Fig. 2.
How does parallel computing arise? For a quantum system of N states we can address up to N independent and
orthogonal initial states. In a system without special selection rules each initial state can evolve into all N final states, Fig.
2b. These N2 paths evolve simultaneously and independently. So our massively parallel computing is performed
concurrently. We are able to read the N2 outputs concurrently because we operate with an ensemble of N state
photoactive structures that are our logic units. There are enough units in the ensemble that fluctuations need not be an
issue. We have demonstrated that we can do this in the laboratory for a solution of bichromophores [1,2] and a paper has
been recently submitted on the details of the experiment and theory.[4] A core objective of COPAC is to now develop this
capability for tailored nanoparticles arrays. Preliminary experimental results (Fig. 3) indicate that this is technologically
feasible. The N2 paths, that provide the output, evolve independently and are distinguishable by being orthogonal.
Perturbations by the environment may in time cause the paths to become numerically linearly dependent. Therefore, in
general the number of independent paths that can be used concurrently can be smaller than N2 and sometimes
significantly smaller, (but it is still larger than N). An important task for computing by observables is to show how to reliably
extract the information carried by most of the N2 paths.

Summary: Long term vision and targeted breakthrough
Our long-term vision is using atomic and molecular time varying processes to implement information processing in
parallel. Within COPAC we have a dense memory provided by the ladder of molecular-states, addressed by broad-band
ultrashort pulses. The dynamic transitions between states implement the logic operations, where the output depends on
the node state when it receives the input, a computational model known as a finite state machine. Using an array built by a
well-tested bottom-up approach we expect to reach a speed of operation that will be faster by at least three orders of
magnitude than achievable by electrical addressing. Data processing will be carried out by compilers that are adapted to
both the hardware and the parallel multivalued logic. Disorder and environment fluctuations are essential for generating
the spatial interference of the emission from different molecules in the ensemble [3]. This will enable an effective interface
with the macroscopic world. In the COPAC fs optical scheme, information is processed before environment induced
decoherence becomes detrimental.
COPAC has four main objectives directed towards enabling the design and the realization of novel high-density and ultrafast parallel logic device at room temperature. The risks associated with these objectives and the means of addressing them are given in Table 3.2b. The objectives for the 4 S/T workpackages are:
Objective 1. To execute dense multivalued parallel logic on a single optically active node in solution
Obj 1 aims to demonstrate and validate the implementation of parallel logic operations on dense level photoactive units and to characterize and benchmark novel photoactive materials.
The challenge here is to achieve at least x10 denser level structure acting in parallel in a single photoactive molecular or nanoparticle complex (‘node’) in solution. This will demonstrate logic design for parallel molecular decision trees and multivalued function transformations based on computing by observables. For each excited electronic state, many vibronic states can be accessed by the broad band laser pulse and will be used for information processing. This number can be very large because several vibrational states of the photoactive node can be resolved and photoactive nodes can be assembled into dimers. For two chromophore dimer a and b with Na and Nb vibronic states respectively, the number of observables is (Na+ Nb)2 = Na 2+ Nb 2+2 NaNb, about twice the size of the two separate systems. The emphasis is on reliably exploiting the dynamics of a high density of vibronic states on each electronic state, with outputs detected as frequency resolved maps in the well-established macroscopic echo direction. This approach will be set up and demonstrated on monomers and dimers of molecular dyes given our previous expertise in the study of their properties. We will then implement the logic schemes on individual quantum dots and dimers and small aggregates thereof in solution. They will provide useful building blocks for the more complex architectures in the arrays (Obj.2 below).
Objective 2: To develop the capability to implement dense multivalued parallel logic on condensed phase arrays.
Obj. 2 expands Obj. 1 to coupled logic units in the solid phase to show that using arrays bypasses the need for concatenating nodes. COPAC will use tailored Semi-conductor Quantum Dots (SC-QD) in quantum confinement regime, organized in self-assembled or layer-by-layer arrays. SC-QDs of different size and/or composition will be engineered by chemical surface functionalization of the nano-objects. The high risk/very high reward aspect here is to resolve a large number of observables in the solid 2D array, which has not yet been achieved. Our preliminary experimental results (see fig.3 and the CV of P2-YP and P3-EC) show that it should be feasible. The main risk is that we will not realize a coherent information transfer between the logic subunits embedded in an array. To explore this we will first use dimers. In any case we will have parallelism within each individual unit and the concatenation of units can be by classical, Foerster-like, resonance transfer known as FRET.
Objective 3. Increasing the number of output directions.
In the nonlinear experimental design the interference between exciting pulses and the molecules in the ensemble leads to a specific pattern of directions in space in which the signal can be read, known as phase matching conditions, PM [3]. We will advance beyond measuring the 2D spectra in the echo direction by measuring several such macroscopic emission directions. Such a detection scheme has not been attempted experimentally before and is a high risk/high reward aspect of this objective. We have done preliminary design work both theoretically and experimentally. From a logic point of view, Obj. 3 will provide an independent way of increasing the density of potential outputs; each phase matching direction corresponds to a different set of logic instructions.
Objective 4. To engineer and validate an integrated module.
It is a target of COPAC that once packaging is achieved in WP4 we seek international standardization and to get it recognized by EPIC-European Photonics Industry Consortium, representing ~300 EU companies in the field, among them some that are very relevant to integrate and manufacture the developed optical-parallel-computing-module.
1.2 Novelty, non-incrementality, plausibility and foundational character
(1) Novelty: COPAC offers a novel foundational approach to massive parallel information processing and a new experimental technology for realizing very dense robust complex parallel logic operations at room temperature.
COPAC will take advantage of the quantum nature of matter on a molecular scale to implement ultrafast high throughput classical parallel and non linear finite state logic. This development, based on the work of Dirac [5] is spelled out in detail in the CV of P2-RL (section 4.1). COPAC will open the way by developing new algorithms, compilers and software based on unconventional hardware.
The coherent quantum dynamics endows the nanosystem with a built-in memory of its history. Because the dynamics of the corresponding Dirac-like classical motion can be proven to be not chaotic, this memory while subject to environmental degradation, is more robust than could be expected. Furthermore, fluctuations induced by the interaction with the environment in the ensemble of addressed nanosystems allow a direct interface with the macroscopic world in several phase matching directions that enables the simultaneous readings of the outputs. Not only the logic is performed in parallel, but the outputs can also be read in parallel.
(2) Foundational character: COPAC provides progress beyond state of the art at several levels. These will enable it to present to European industries an optical-parallel-computing-module that is ready for manufacturing and COPAC 5 implementation of massive data handling: First and foremost COPAC will provide classical multivalued massively parallel logic with capabilities in principal similar to quantum computing [6] and that can be realistically implemented in the laboratory. This is made possible by our quantum engineering of a system that allows for a classical-like computing by observables. These observables are defined on both vibrational (or phonon) and electronic degrees of freedom to produce an unprecedented dense logic. In two distinct ways COPAC will realize unprecedented massive parallelism. One by taking advantage of multi-phase-matching configuration that will be able to provide very dense information content of the response outputs. The other by seeking to couple individual photoactive units (nodes) on an array so as to increase the density of inputs. Special synthetic and characterization efforts will be devoted to preparing novel photoactive elements. COPAC will also make key advances in the foundational scheme of molecular logic units by going beyond the perturbation theory limit description of how to perturb (= address) a system thereby enhancing parallelism. The potential of the new advanced hardware will be exploited by the development and realization of new compilers and software packages adapted to the novel parallel capabilities of the hardware. Lastly but very important is that COPAC will build upon the very impressive current progress in functionalized nanostructures and nanooptics. COPAC will go well beyond the current state of the art by endowing the units with multivalued parallel logic functionality. In making this progress COPAC will explore new materials, characterize their spectroscopic response and develop quantum chemistry modelling and quantum tomography for computing by observables towards implementing parallel multichromophoric logic.
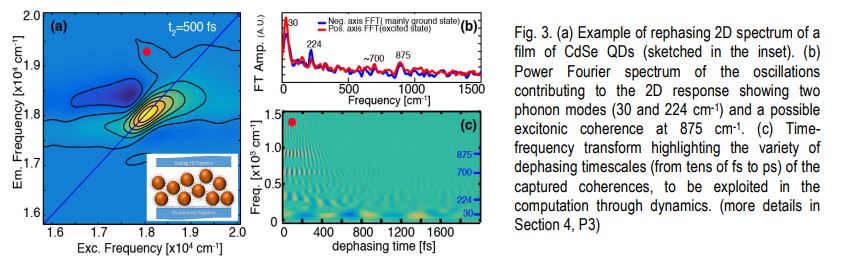
(3) Plausibility of the proposed research has been explored by our preliminary experiments and detailed theory. In particular we have demonstrated the operation of parallel logic for experimental data on bichromophoric system in solution [2]. This also demonstrated coherent energy transfer between the two chromophores that survives for many vibrations [4]. Most recently, unpublished, we have clear experimental results of a macroscopic readout of 2D spectroscopy on arrays of quantum dots, Fig. 3. Earlier detailed theoretical studies showed how to implement parallel logic [1] and how to perform massively parallel logic by macroscopic reading in different phase matching directions [3].
1.3 Workplan
Methodology. COPAC is organized as four tightly interlocking WP’s involving a joint effort of the partners to make synergetic progress towards a more and more dense and complex parallel logic and software development that take advantage of the unconventional hardware Each WP has an interdisciplinary character so as to ensure swift corrective measures if and as needed.
WP1 : Theory, modelling, logic design, and software and compiler developments ensures a direct link between the physics of the hardware and compilers. It is lead by Prof. Raphael D. Levine from HUJI (P2), with Prof. Françoise Remacle from ULg (P1), Dr. Barbara Fresch from UNIPD (P3) and David Herrera-Marti and Emmanuel Mazer from Probayes (P6) as partners.
WP2: Design, synthesis and characterization of quantum dot arrays gathers synthesis of SC-QD and their assembly, as well as their characterization towards controlled interunit electronic couplings, which is essential to the project. It is led by Dr. Marinella Striccoli from CNR-IPCF (P4) with Prof. Yossi Paltiel from HUJI (P2) as partner
WP3: Parallel logic by non linear optics is the non-linear optics multiphase directions experiments. It is lead by Prof. Elisabetta Collini from UNIPD (P3)
WP4: Device Engineering is the modelling and packaging the device towards industrial stakeholders. WP4 will lead the way to building of a strong community based on parallel optical computing. It is led by Dr. Ariela Donval with Dr. Moshe Oron from KiloLambda (P5).
WP5 ensures an effective management and the flow of information and results between the RTD WP’s, and is in charge of exploitation and dissemination. It is led by Françoise Remacle from ULg (P1).
Organization and timing of tasks
1.4 Expertise of the partners
Participant # |
Partner Name & Expertize |
WP |
P1-FR ULg |
Francoise Remacle, Quantum Theory, Dynamics & Molecular logic |
1,3,5 |
P2-RL HUJI |
Raphael D Levine, Quantum Dynamics & Logic Synthesis |
1,3,5 |
P2-YP HUJI |
Yossi Paltiel, Quantum Engineering of NP arrays and Optical Addressing & Readout |
2,3,5 |
P3-EC UNIPD |
Elisabetta Collini, Multidimensional Spectroscopy |
3,4,5 |
P3-BF UNIPD |
Barbara Fresch, Simulation and Modelling of Logic Units |
1,3,5 |
P4-MS CNR |
Marinella Striccoli, Design and Synthesis of Nanoparticles and their Assembly |
2,3,4,5 |
P5-MO, AR,NG KL |
Moshe Oron, Ariela Donval, Noam Gross Device Engineering, testing and packaging |
2,3,4,5 |
P6-EM, DHM, Probayes |
Emmanuel Mazer, David Herrera-Marti, Software and compilers for Unconventional Hardware |
1,3,4,5 |
|
|
 |
|
 |
|